Work underway in the WPI laboratory of Ulkuhan Guler, assistant professor of electrical engineering, aims to change all that by developing a patch with miniaturized sensors and electronics that can continuously collect all of the critical measures of respiration—something no other single device can do. Worn on the skin like a Band-Aid, the patch could be used in hospitals and clinics, and even by patients in their homes. By transmitting data to physicians wirelessly, it could provide an early warning of potentially serious complications.
Ancient Greeks thought all diseases were the result of imbalances in four bodily fluids, or humors: yellow bile, black bile, phlegm, and blood. Medical practice has advanced greatly since then, but when it comes to understanding and diagnosing disorders of the cardiovascular system, modern doctors still look to a small set of vital signs: pulse, blood pressure, respiration rate, the partial pressure of oxygen and carbon dioxide in the arteries, oxygen saturation, and the total oxygen content of the blood. Taken together, these parameters can indicate whether the heart and lungs are functioning normally—and if they aren’t, they can show what the source of the malfunction might be.
Currently, clinicians must use multiple sampling methods to obtain this information. For example, oxygen saturation (the ratio of how much oxygen is in the blood versus how much it can normally hold) can be measured with a pulse oximeter, a device that clips to the end of a finger. But pulse oximeters can’t detect when blood is oversaturated, a condition called hyperoxemia, which sometimes occurs when patients are given supplemental oxygen. In adults, hyperoxemia can lead to oxygen poisoning, which can damage the lungs and other organs; in infants, it can injure the retina and white and gray matter in the brain, contributing to blindness and cognitive impairment.
The most reliable way of measuring partial arterial pressure of oxygen and carbon dioxide, which indicate how well these gases are moving between the lungs and the blood, involves an arterial blood draw, a painful procedure. Even in intensive care units, samples are taken infrequently—often just three times a day—which means dramatic changes can go undetected for hours. Devices exist that can measure these blood gases noninvasively, but the technology is bulky and expensive, and some units use heaters to increase the perfusion of gases through the skin, causing burns if the sensors are not moved frequently.
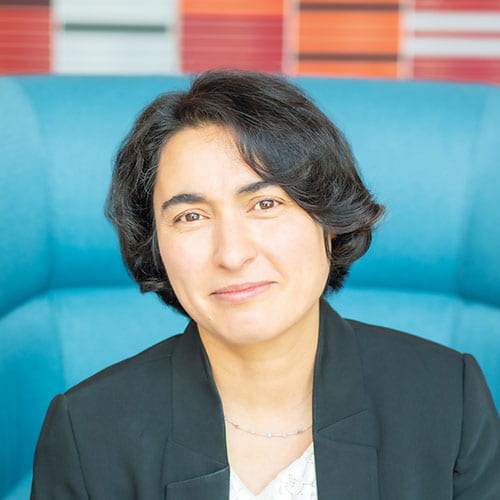
Ulkuhan Guler
Four years into her work toward this ambitious objective, Guler and her research team still have many challenges to overcome. But their progress to date, made possible in part by more than $1 million in federal and corporate funding, makes her optimistic that her vision of an all-in-one respiratory sensor patch could be realized in the not-too-distant future.
Finding a Niche
Guler, who earned a BS in electronics and communication at Istanbul Technical University, an MS in electronics engineering at the University of Tokyo, and a PhD in electronics engineering at Boğaziçi University in Istanbul, joined the WPI faculty in 2018 after three years as a postdoctoral research fellow in electronics engineering at Georgia Tech, where, among other accomplishments, she developed an application-specific chip that provides wireless power and data for implantable medical devices.
“By training, I am an electronics engineer,” she says. “I design electronic chips. But I am also interested in doing biomedical research, because the next generation of biomedical devices will be smart, miniaturized, wearable, and implantable.” Which means they will be driven by chips.
Ulkuhan GulerThe next generation of biomedical devices will be smart, miniaturized, wearable, and implantable.
Early in her time at WPI, she learned that new researchers should seek out a focus area that builds on their previous work, but one that also blazes a new path. In search of her niche, she asked doctors at the UMass Memorial Health system what kinds of biomedical technology they most urgently needed. Among them was Lawrence M. Rhein, MD, chief of the UMass Memorial Division of Neonatology and chair of the Department of Pediatrics.
“He told me about the importance of finding a better way to measure blood gases,” she says. “Little babies don’t have as much blood to lose as older children and adults, and when blood is taken, the data is only of the moment. But doctors really want to observe the history.” And even when noninvasive means are used to monitor infants with respiratory distress, they must be tethered to a device in the neonatal intensive unit (NICU), sometimes for days—as Guler knows from personal experience.
Fourteen years ago, she delivered her first child, Musa, in a hospital in Istanbul. “They gave him to me for an hour,” she says, “and then they noticed that something was wrong with his breathing.” She stayed in the hospital for three days while her newborn son was hooked up to monitors in the NICU. “I could hold him for only 10 or 15 minutes at a time. I wanted to be close to him; it was so frustrating.”
Guler soon had a mission and a research partner, as Rhein has been a collaborator on all the work done to date. “We consult him about the devices we are developing, about our experimental results, about anything related to medicine,” she says. Guler is also collaborating with Bige Deniz Unluturk, assistant professor of electrical engineering and biomedical engineering at Michigan State University, an expert on computational modeling in biomedical engineering, and with John McNeill, WPI’s Bernard M. Gordon Dean of Engineering and an expert on integrated circuits, who hired Guler when he was head of the Department of Electrical Engineering.
Early Breakthroughs
Developing new biomedical devices is not only technically challenging, it’s expensive. So having found her niche, Guler began applying for funding. Money from the WPI-UMass Chan Medical School Collaborative Seed Funding Grant Program and two awards from Analog Devices, a semiconductor company in Wilmington, Mass., helped equip her lab and launch work on the development of a transcutaneous oxygen sensor. (Rhein also lent Guler instruments she uses for testing.)
The research program received a major boost with two awards from the National Science Foundation: a $500,000 award for developing computational models for a wearable blood gas monitor for infants, and a five-year, $500,000 CAREER award to fund work on a noninvasive miniaturized blood gas sensor for respiration monitoring. CAREER awards are the NSF’s most prestigious awards for young faculty members seeking to establish new research programs.
Guler and her team also did their homework. For nearly six months they read everything they could find on respiration, blood gases, and methods for measuring them. “We wanted to understand,” she says, “what kind of sensing technologies are available and suitable for this research, for miniaturization, and for being used in a medical device.” The team, which included Rhein and Guler’s first two PhD students, Ian Costanzo and Devdip Sen, summarized their findings in a heavily cited paper in the journal IEEE Reviews in Biomedical Engineering.
The team learned that oxygen that diffuses through the skin can be directly correlated with the partial pressure of oxygen in the arteries—what existing noninvasive devices measure. Guler and her team, which now includes four PhD candidates, two master’s students, and teams of undergraduates completing Major Qualifying Projects, decided to try to measure diffused oxygen and carbon dioxide with luminescence films, which respond to light of specific frequencies. By shining blue light on one type of luminescence film, they could get it to emit red light, which is absorbed by oxygen molecules. Since the amount of red light bouncing back to the sensor would be inversely proportional to the amount of oxygen present, the intensity of the reflected light should indicate the quantity of diffused oxygen.

The Guler Lab
Guler’s team produced a series of oxygen sensor prototypes. “With the first prototype, we could see that we could sense high and low oxygen in a test chamber,” she says. “That was really so exciting. We knew it was possible, but it was the first time we had seen it with our own eyes.”
Through such laboratory experiments, they learned that monitoring intensity alone would not produce reliably accurate measurements. Better results could be obtained by capturing both intensity and decay time, a measure of how long it takes for the intensity of the reflected light to drop below a preset level. With these results in hand and the oxygen sensor advancing well, Guler has turned her attention to measuring carbon dioxide, a far more difficult nut to crack.
Similar to the oxygen sensor, the carbon dioxide sensor will use light-emitting material. She has chosen a fairly new type of fluorescence film, called a fluorophore film, because it can be integrated with the oxygen sensor in the same device. Again, as with the oxygen sensor, Guler proposes to measure both the intensity of light and the decay time. “Measuring decay time for oxygen is relatively easy,” she says, “because it is slow—in the microsecond range. But for carbon dioxide, it is in the low nanosecond range, and that is very difficult to capture.”
Guler says she and her students are exploring methods beyond decay time for obtaining accurate CO2 readings, including measuring tiny differences in the phase of the transmitted and reflected light. They are also exploring whether the ratio of intensity and decay time may provide better information than either factor alone. “The reading method is everything.” she says. “Otherwise, we may be reading junk.”
Refining and Dreaming
To pack all of the components of a wearable respiration sensor into a Band-Aid–sized patch, everything needs to be small. That begins with the two luminescence film sensors for oxygen and carbon dioxide, which will be about the size and shape of the holes made by a paper hole punch. The patch will also include tiny light-emitting diodes and photodiodes for generating and detecting light, circuitry for wireless data transmission, a power source (batteries or wireless power transfer), and a tiny chip.
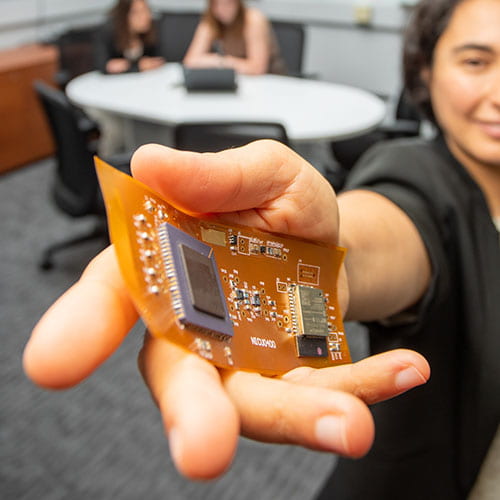
Integrated into the chip will be custom-designed algorithms for calibrating the sensors and processing the data they produce. Among these algorithms will be computational models that will adjust the sensor data to compensate for factors that can affect the permeability of skin, and thus the ability of oxygen and carbon dioxide to diffuse through it, including age, sex, height, and weight.
The team will also explore ways to make the patch comfortable to wear for up to a week. This and other aspects of the design will be refined through tests in the lab and, ultimately, through human trials. When the research reaches that critical point, Guler says she will likely explore partnerships with biomedical device manufacturers and also seek funding from the National Institutes of Health, which can support trials with human volunteers and actual patients. She says she will also look to Rhein for his expertise in this phase of the work.
While her focus now is on fulfilling the goals of her current NSF funding, which is limited to the miniaturized, wearable arterial oxygen and carbon dioxide sensor patch, Guler is also looking to future challenges. She says she believes the work her lab is undertaking can be extended to incorporate the other critical aspects of respiration that will help doctors diagnose and track respiratory ailments. “What I am dreaming of is a complete respiration patch,” she says. “So, we will be looking to combine the two parameters we are currently working to measure with things like oxygen saturation, respiration rate, and total blood oxygen content. This is work that will stretch beyond the five years of my CAREER Award.”
She is also thinking about the applications such a patch would make possible (beyond monitoring patients in the clinic and at home). She says she has already received interest in her research from the U.S. military, which would like to be able to wirelessly monitor the respiration of soldiers stationed at high altitudes. Guler also envisions incorporating her respiration monitor into smart watches and fitness monitors, to give healthy people more useful data on the state of their hearts and lungs. And then there are the mounds of data that a continuously operating respiration patch could produce, data that could be a boon to research and public health.
For example, she wonders whether our understanding of COVID-19 and its effect on respiration might have become clear earlier had a patch like hers been in widespread use. And she says she would like to collaborate with Rhein on researching the role of oxygen in fetal and infant development, and on the risks of administering supplemental oxygen to babies, something her technology could make possible.
But most of all, she says, she is motivated by the quest to build technology that can make life better for people around the world. “If we can create this device and make it affordable,” she says, “it could make a difference for the whole of humanity. It would be so rewarding to see the technology that we develop become useful for so many people from so many countries and backgrounds.”